How Cells Swallow
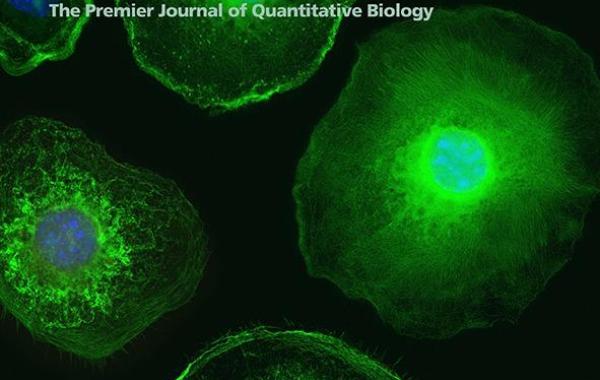
Cells have no obvious entryways for materials around them. They bring things inside by engulfing objects. Immune cells, for example, engulf pathogens, which can be larger than themselves. The process is called phagocytosis.
Phagocytosis depends on a cascade of chemical signals that instruct the cell to envelop the target and form a space where the pathogen will eventually be destroyed. Using super-resolution imaging, a Georgia Tech research team has observed the molecular reorganizations involved in the process. The study was published in and featured on the cover of the December 20, 2016, issue of Biophysical Journal.
The findings advance the understanding of how immune cells destroy pathogens, which could lead to new ways to treat infectious diseases.
“It was well-known that changes in a cell’s shape during phagocytosis are primarily driven by the molecular reorganization of actin,” says Daniel T. Kovari, the lead author of the study. Kovari is a former Ph.D. student in the School of Physics who worked with Associate Professor Jennifer E. Curtis. Research in the Curtis lab focuses on mechanobiology and biointerfaces.
Actin is a protein involved in mechanically directing cell motion and is part of the cell’s cytoskeleton, the molecular network that gives structure to cells. “It was known that actin filaments aggregate near the region where a cell has made contact with a pathogen,” says Kovari, who is now a postdoctoral fellow at Emory University.
“It was unclear how those filaments arrange during phagocytosis,” Curtis says. “We were particularly confused about how actin filaments can drive cell membrane extension around the target pathogen and then eventually constrict like a belt to pull the particle inward. The two processes require two very different orientations of the filaments. We set out to resolve how this could happen.”
To visualize the process, the researchers used Georgia Tech’s capability in super-resolution structured illumination optical microscopy, housed in the Optical Microscopy Core at the Parker H. Petit Institute for Bioengineering and Bioscience.
“We captured detailed images of the actin cytoskeleton in white blood cells as they went through a flattened version of phagocytocis,” Kovari says.
OBSERVING PHAGOCYTOCIS
Phagocytosis occurs in three-dimensional space, but microscopic techniques are two-dimensional. To overcome this mismatch, the team flattened the experimental set-up.
“Instead of presenting simulated pathogen particles to white blood cells, we deposited the cells onto a microscope slide coated with antibodies, tricking the cells to perceive the entire surface as a giant pathogen,” Kovari says. “Once the cells make contact with the slide, they spread out, as if trying to engulf the entire surface, enabling us to capture the fine details of actin organization during phagocytosis.”
Phagocytosis is an intricate process. Much research has focused on teasing out details of the chemical signaling cascade that triggers and orchestrates pathogen engulfment, Kovari says. The biophysics aspects—How does the cell change shape? What forces does the cell exert?—have not received as much attention.
“But even in biophysics studies, phagocytic behavior is traditionally assayed by presenting white blood cells with pathogen-like particles,” Kovari notes. “While that method faithfully simulates natural phagocytosis, it is inherently three-dimensional and not suited for study using super-resolution microscopy, which works best when imaging a single plane. Without the added clarity achieved through super-resolution imaging, precise organization of actin fibers could not be discerned.”
ACTIN ORGANIZATION DURING PHAGOCYTOSIS
Super-resolution imaging revealed that actin arranges into two motifs during phagocytosis. As the cell is surrounding its target, actin forms a dense zone at the leading edges of the cell, expanding the zone and applying pressure to the cell membrane, pushing it out and around the target.
When the cell is stretched over its target, it pinches down and pulls the target into its interior. During this second phase, actin dramatically reorganizes to forms fiber-like bundles around the target, parallel to the cell membrane. Like purse strings, these bundles are pulled tight by molecular motor proteins, driving the membrane to close and trapping the pathogen inside.
Coauthors of the study are Wenbin Wei, Patrick Chang, Jan-Simon Toro, Ruth Fogg Beach, Karen Porter, Doyeon Koo, and Jennifer E. Curtis, from the Georgia Tech School of Physics, and Dwight Chambers, from the Georgia Tech Wallace H. Coulter Department of Biomedical Engineering.
This study was supported by grants from the National Science Foundation: PHY-0848797 and SRN-POLS 1205878.
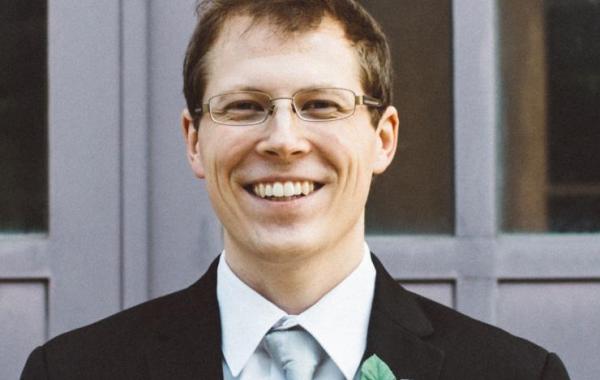
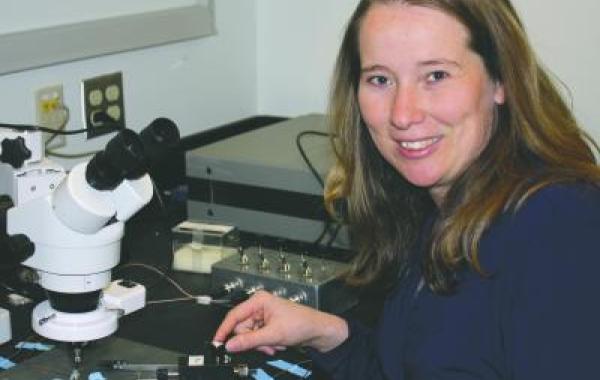